555 timer IC
An integrated circuit chip for timing applications
The 555 timer IC is an integrated circuit (chip) used in a variety of timer, delay, pulse generation, and oscillator applications. Derivatives provide two (556) or four (558) timing circuits in one package.[2] The design was first marketed in 1972 by Signetics.[3][4] Since then, numerous companies have made the original bipolar timers, as well as similar low-power CMOS timers. In 2017, it was said that over a billion 555 timers are produced annually by some estimates, and that the design was "probably the most popular integrated circuit ever made".[5]
History [ edit ]
Silicon die of the first 555 chip (1971)
Die of a CMOS NXP ICM7555 chip
The timer IC was designed in 1971 by Hans Camenzind under contract to Signetics.[3] In 1968, he was hired by Signetics to develop a phase-locked loop (PLL) IC. He designed an oscillator for PLLs such that the frequency did not depend on the power supply voltage or temperature. Signetics subsequently laid off half of its employees due to the 1970 recession, and development on the PLL was thus frozen.[6] Camenzind proposed the development of a universal circuit based on the oscillator for PLLs and asked that he develop it alone, borrowing equipment from Signetics instead of having his pay cut in half. Camenzind's idea was originally rejected, since other engineers argued the product could be built from existing parts sold by the company; however, the marketing manager approved the idea.[7]
The first design for the 555 was reviewed in the summer of 1971. Assessed to be without error, it proceeded to layout design. A few days later, Camenzind got the idea of using a direct resistance instead of a constant current source, finding that it worked satisfactorily. The design change decreased the required 9 external pins to 8, so the IC could be fit in an 8-pin package instead of a 14-pin package. This revised version passed a second design review, and the prototypes were completed in October 1971 as the NE555V (plastic DIP) and SE555T (metal TO-5).[8] The 9-pin version had already been released by another company founded by an engineer who had attended the first review and had retired from Signetics; that firm withdrew its version soon after the 555 was released. The 555 timer was manufactured by 12 companies in 1972, and it became a best-selling product.[6]
Name [ edit ]
Several books report the name "555" derived from the three 5 kΩ resistors inside the chip.[9][10][11] However, in a recorded interview with an online transistor museum curator,[12] Hans Camenzind said "It was just arbitrarily chosen. It was Art Fury [Marketing Manager] who thought the circuit was gonna sell big who picked the name '555'."[13]
Design [ edit ]
Depending on the manufacturer, the standard 555 package incorporated the equivalent of 25 transistors, 2 diodes, and 15 resistors on a silicon chip packaged into an 8-pin dual in-line package (DIP-8).[14][15] Variants available included the 556 (a DIP-14 combining two complete 555s on one chip),[16] and 558 / 559 (both variants were a DIP-16 combining four reduced-functionality timers on one chip).[2]
The NE555 parts were commercial temperature range, 0 °C to +70 °C, and the SE555 part number designated the military temperature range, −55 °C to +125 °C. These chips were available in both high-reliability metal can (T package) and inexpensive epoxy plastic (V package) form factors. Thus, the full part numbers were NE555V, NE555T, SE555V, and SE555T.
Low-power CMOS versions of the 555 are now available, such as the Intersil ICM7555 and Texas Instruments LMC555, TLC555, TLC551.[17][18] [19][20]
Internal schematic [ edit ]
The internal block diagram and schematic of the 555 timer are highlighted with the same color across all three drawings to clarify how the chip is implemented:[2]
Voltage Divider : Between the positive supply voltage V CC and the ground GND is a voltage divider consisting of three identical resistors, which create two reference voltages at 1 ⁄ 3 V CC and 2 ⁄ 3 V CC . The latter is connected to the "Control" pin. All three resistors have the same resistance, 5 kΩ for bipolar timers, 100 kΩ (or higher) for CMOS timers.
: Between the positive supply voltage V and the ground GND is a voltage divider consisting of three identical resistors, which create two reference voltages at V and V . The latter is connected to the "Control" pin. All three resistors have the same resistance, 5 kΩ for bipolar timers, 100 kΩ (or higher) for CMOS timers. Threshold Comparator : The comparator's negative input is connected to the higher reference voltage divider of 2 ⁄ 3 V CC (and "Control" pin), and the comparator's positive input is connected to the "Threshold" pin.
: The comparator's negative input is connected to the higher reference voltage divider of V (and "Control" pin), and the comparator's positive input is connected to the "Threshold" pin. Trigger Comparator : The comparator's positive input is connected to the lower reference voltage divider of 1 ⁄ 3 V CC , and the comparator's negative input is connected to the "Trigger" pin.
: The comparator's positive input is connected to the lower reference voltage divider of V , and the comparator's negative input is connected to the "Trigger" pin. Flip-Flop : An SR flip-flop stores the state of the timer and is controlled by the two comparators. The "Reset" pin overrides the other two inputs, thus the flip-flop (and therefore the entire timer) can be reset at any time.
: An SR flip-flop stores the state of the timer and is controlled by the two comparators. The "Reset" pin overrides the other two inputs, thus the flip-flop (and therefore the entire timer) can be reset at any time. Output : The output of the flip-flop is followed by an output stage with push-pull (P.P.) output drivers that can load the "Output" pin with up to 200 mA for bipolar timers, lower for CMOS timers.
: The output of the flip-flop is followed by an output stage with push-pull (P.P.) output drivers that can load the "Output" pin with up to 200 mA for bipolar timers, lower for CMOS timers. Discharge : Also, the output of the flip-flop turns on a transistor that connects the "Discharge" pin to the ground.
555 internal block diagram [1]
555 internal schematic of bipolar version
555 internal schematic of CMOS version
Pinout [ edit ]
The pinout of the 8-pin 555 timer[1] and 14-pin 556 dual timer[21] are shown in the following table. Since the 556 is conceptually two 555 timers that share power pins, the pin numbers for each half are split across two columns.[2]
In the following table, longer pin designations are used, because manufacturers never standardized the abbreviated pin names across all datasheets.
555 pin# 556:1st pin# 556:2nd pin# Pin name Pin direction Pin description[1][21][2] 1 7 7 GND Power Ground supply: this pin is the ground reference voltage (zero volts).[22] 2 6 8 TRIGGER Input Trigger: when the voltage at this pin falls below 1 ⁄ 2 of the voltage of CONTROL ( 1 ⁄ 3 V CC , except when CONTROL is driven by an external signal), the OUTPUT goes to the high state and a timing interval starts.[22] As long as this pin continues to be kept at a low voltage, the OUTPUT will remain in the high state. 3 5 9 OUTPUT Output Output: this pin is a push-pull (P.P.) output that is driven to either a low state (GND) or a high state (for bipolar timers, V CC minus approximately 1.7 volts) (for CMOS timers, V CC ). For bipolar timers, this pin can drive up to 200 mA, but CMOS timers are able to drive less (varies by chip). For bipolar timers, if this pin drives an edge-sensitive input of a digital logic chip, a 100 to 1000 pF decoupling capacitor (between this pin and GND) may need to be added to prevent double triggering.[2] 4 4 10 RESET Input Reset: a timing interval may be reset by driving this pin to GND, but the timing does not begin again until this pin rises above approximately 0.7 volts. This pin overrides TRIGGER , which in turn overrides THRESHOLD. If this pin is not used, it should be connected to V CC to prevent electrical noise accidentally causing a reset.[23][22] 5 3 11 CONTROL Input Control: this pin provides access to the internal voltage divider ( 2 ⁄ 3 V CC by default). By applying a voltage to this pin, the timing characteristics can be changed. In astable mode, this pin can be used to frequency-modulate the OUTPUT state. If this pin is not used, it should be connected to a 10 nF decoupling capacitor (between this pin and GND) to ensure electrical noise doesn't affect the internal voltage divider.[2][23][22] 6 2 12 THRESHOLD Input Threshold: when the voltage at this pin is greater than the voltage at CONTROL ( 2 ⁄ 3 V CC except when CONTROL is driven by an external signal), then the OUTPUT high state timing interval ends, causing the OUTPUT to go to the low state.[22] 7 1 13 DISCHARGE Output Discharge: For bipolar timers, this pin is an open-collector (O.C.) output, CMOS timers are open-drain (O.D.). This pin can be used to discharge a capacitor between intervals, in phase with the OUTPUT. In bistable mode and schmitt trigger mode, this pin is unused, which allows it to be used as an alternate output.[22] 8 14 14 V CC Power Positive supply: For bipolar timers, the voltage range is typically 4.5 to 16 volts, some are spec'ed for up to 18 volts, though most will operate as low as 3 volts. For CMOS timers, the voltage range is typically 2 to 15 volts, some are spec'ed for up to 18 volts, and some are spec'ed as low as 1 volt. See the supply min and max columns in the derivatives table in this article. Decoupling capacitor(s) are generally applied (between this pin and GND) as a good practice.[24][23]
Pinout of 555 single timer. [1] [2]
Pinout of 556 dual timer.[21][2]
Modes [ edit ]
The 555 IC has the following operating modes:
Astable (free-running) mode – The 555 can operate as an electronic oscillator. Uses include LED and lamp flashers, pulse generation, logic clocks, tone generation, security alarms, pulse-position modulation, and so on. The 555 can be used as a simple ADC, converting an analog value to a pulse length (e.g., selecting a thermistor as timing resistor allows the use of the 555 in a temperature sensor with the period of the output pulse determined by the temperature). The use of a microprocessor-based circuit can then convert the pulse period to temperature, linearize it, and even provide calibration means. Monostable (one-shot) mode – In this mode, the 555 functions as a "one-shot" pulse generator. Applications include timers, missing pulse detection, bounce-free switches, touch switches, frequency dividers, capacitance measurement, pulse-width modulation (PWM), and so on. Bistable (flip-flop) mode – The 555 operates as an SR flip-flop. Uses include bounce-free latched switches. Schmitt trigger (inverter) mode – the 555 operates as a Schmitt trigger inverter gate which converts a noisy input into a clean digital output.
Astable [ edit ]
Schematic of a 555 timer in astable mode
Waveform in astable mode
Astable mode examples with common values Frequency C R 1 R 2 Duty cycle 0.1 Hz (+0.048%) 100 µF 8.2 kΩ 68 kΩ 52.8% 1 Hz (+0.048%) 10 µF 8.2 kΩ 68 kΩ 52.8% 10 Hz (+0.048%) 1 µF 8.2 kΩ 68 kΩ 52.8% 100 Hz (+0.048%) 100 nF 8.2 kΩ 68 kΩ 52.8% 1 kHz (+0.048%) 10 nF 8.2 kΩ 68 kΩ 52.8% 10 kHz (+0.048%) 1 nF 8.2 kΩ 68 kΩ 52.8% 100 kHz (+0.048%) 100 pF 8.2 kΩ 68 kΩ 52.8%
In the astable configuration, the 555 timer puts out a continuous stream of rectangular pulses having a specific frequency. The astable configuration is implemented using two resistors, R 1 {displaystyle R_{1}} and R 2 {displaystyle R_{2}} , and one capacitor C {displaystyle C} . In this configuration, the control pin is not used, thus it is connected to ground through a 10 nF decoupling capacitor to shunt electrical noise. The threshold and trigger pins are connected to the capacitor C {displaystyle C} ; thus they have the same voltage.
Initially, the capacitor C {displaystyle C} is not charged, thus the trigger pin receives zero voltage, which is less than 1⁄3 of the supply voltage. Consequently, the trigger pin causes the output to go high and the internal discharge transistor to go to cut-off mode. Since the discharge pin is no longer short-circuited to ground, the current flows through the resistors R 1 {displaystyle R_{1}} and R 2 {displaystyle R_{2}} to the capacitor, charging it. The capacitor C {displaystyle C} starts charging until the voltage becomes 2⁄3 of the supply voltage.
At that time, the threshold pin causes the output to go low and the internal discharge transistor to go into saturation mode. Consequently, the capacitor starts discharging through R 2 {displaystyle R_{2}} until it becomes less than 1⁄3 of the supply voltage, at which point the trigger pin causes the output to go high and the internal discharge transistor to go to cut-off mode once again. And the cycle repeats.
During the first pulse, the capacitor charges from zero to 2⁄3 of the supply voltage, however, in later pulses, it only charges from 1⁄3 to 2⁄3 of the supply voltage. Consequently, the first pulse has a longer high time interval compared to later pulses. Moreover, the capacitor charges through both resistors but only discharges through R 2 {displaystyle R_{2}} , thus the output high interval is longer than the low interval. This is shown in the following equations:
The output high time interval of each pulse is given by:
t high = ln ( 2 ) ⋅ ( R 1 + R 2 ) ⋅ C {displaystyle t_{text{high}}=ln(2)cdot (R_{1}+R_{2})cdot C}
The output low time interval of each pulse is given by:
t low = ln ( 2 ) ⋅ R 2 ⋅ C {displaystyle t_{text{low}}=ln(2)cdot R_{2}cdot C}
Hence, the frequency f {displaystyle f} of the pulse is given by:[25]
f = 1 t high + t low = 1 ln ( 2 ) ⋅ ( R 1 + 2 R 2 ) ⋅ C {displaystyle f={frac {1}{t_{text{high}}+t_{text{low}}}}={frac {1}{ln(2)cdot (R_{1}+2R_{2})cdot C}}}
and the duty cycle (%) is given by:
duty % = t high t high + t low ⋅ 100 = R 1 + R 2 R 1 + 2 R 2 ⋅ 100 {displaystyle {text{duty}}%={frac {t_{text{high}}}{t_{text{high}}+t_{text{low}}}}cdot 100={frac {R_{1}+R_{2}}{R_{1}+2R_{2}}}cdot 100}
where t {displaystyle t} is the time in seconds, R {displaystyle R} is the resistance in ohms, C {displaystyle C} is the capacitance in farads, and ln ( 2 ) {displaystyle ln(2)} is the natural logarithm of 2 (a constant which is 0.693147 when rounded to 6 significant digits), but it is commonly approximated with fewer digits in 555 timer books and datasheets, such as 0.7, 0.69, or 0.693.
Schematic of a 555 timer in astable mode with a 1N4148 diode to create a duty cycles less than 50%
Resistor R 1 {displaystyle R_{1}} requirements:
W {displaystyle W} R 1 {displaystyle R_{1}} V CC ⋅ V CC R 1 {displaystyle {frac {V_{text{CC}}cdot V_{text{CC}}}{R_{1}}}} Ohm's law.
Ohm's law. Particularly with bipolar 555s, low values of R 1 {displaystyle R_{1}}
The first cycle will take appreciably longer than the calculated time, as the capacitor must initially charge from 0 V to 2⁄3 of V CC from power-up, but only from 1⁄3 of V CC to 2⁄3 of V CC on subsequent cycles.
Shorter duty cycle [ edit ]
To create an output high time shorter than the low time a duty cycle less than 50%) a fast diode 1N4148 signal diode) can be placed in parallel with R 2 , with the cathode on the capacitor side. This bypasses R 2 during the high part of the cycle, so that the high interval depends only on R 1 and C, with an adjustment based the voltage drop across the diode. The voltage drop across the diode slows charging on the capacitor, so that the high time is longer than the expected and often-cited ln(2)⋅R 1 C = 0.693 R 1 C. The low time will be the same as above, 0.693 R 2 C. With the bypass diode, the high time is:
t high = ln ( 2 V CC − 3 V diode V CC − 3 V diode ) ⋅ R 1 ⋅ C , {displaystyle t_{text{high}}=ln left({frac {2V_{text{CC}}-3V_{text{diode}}}{V_{text{CC}}-3V_{text{diode}}}}right)cdot R_{1}cdot C,}
where V diode is when the diode's "on" current is 1⁄2 of V CC /R 1 , which can be determined from its datasheet or by testing. As an extreme example, when V CC = 5 V, and V diode = 0.7 V, high time is 1.00 R 1 C, which is 45% longer than the "expected" 0.693 R 1 C. At the other extreme, when V cc = 15 V, and V diode = 0.3 V, the high time is 0.725 R 1 C, which is closer to the expected 0.693 R 1 C. The equation reduces to the expected 0.693 R 1 C if V diode = 0 V.
Monostable [ edit ]
Schematic of a 555 in monostable mode. Example values R = 220 kΩ, C = 100 nF for debouncing a pushbutton.
Waveform in monostable mode
In monostable mode, the output pulse ends when the voltage on the capacitor equals 2⁄3 of the supply voltage. The output pulse width can be lengthened or shortened to the need of the specific application by adjusting the values of R and C.[26]
The output pulse is of width t, which is the time it takes to charge C to 2⁄3 of the supply voltage. It is given by:
t = ln ( 3 ) ⋅ R ⋅ C , {displaystyle t=ln(3)cdot Rcdot C,}
where t {displaystyle t} is the time in seconds, R {displaystyle R} is the resistance in ohms, C {displaystyle C} is the capacitance in farads, ln ( 3 ) {displaystyle ln(3)} is the natural log of 3 constant, which is 1.098612 (rounded to 6 significant digits), but it is commonly rounded to fewer digits in 555 timer books and datasheets, like 1.1 or 1.099.
While using the timer IC in monostable mode, the time span between any two triggering pulses must be greater than the RC time constant.[27]
Examples [ edit ]
Monostable mode examples with common values Time C R 100 µs (−0.026%) 1 nF 91 kΩ 1 ms (−0.026%) 10 nF 91 kΩ 10 ms (−0.026%) 100 nF 91 kΩ 100 ms (−0.026%) 1 µF 91 kΩ 1 s (−0.026%) 10 µF 91 kΩ 10 s (−0.026%) 100 µF 91 kΩ
Using the algebraic timing formula (above) and component values from the example table (right), time is calculated as follows:
t = ln ( 3 ) ⋅ R ⋅ C {displaystyle t=ln(3)cdot Rcdot C}
when R is 91 kΩ and C is 100 nF
t = ln ( 3 ) ⋅ 91 k ⋅ 100 n {displaystyle t=ln(3)cdot 91kcdot 100n}
is converted into the expected units: ln ( 3 ) {displaystyle ln(3)} is natural log of 3, R {displaystyle R} is resistance in ohms, C {displaystyle C} is capacitance in farads,
t = 1.098612 ⋅ 91000 ⋅ 0.0000001 {displaystyle t=1.098612cdot 91000cdot 0.0000001}
is multiplied together
t = 0.0099973692 {displaystyle t=0.0099973692}
Using algebraic math, component values can be scaled by powers of 10 to get the same timing:
10 ms (−0.026%) = 10 nF and 910 kΩ
10 ms (−0.026%) = 100 nF and 91 kΩ : (values from table)
10 ms (−0.026%) = 1000 nF and 9.1 kΩ : (1000 nF is 1 µF)
For each row in the example table (right), two additional timing values can easily be created by adding a second resistor in parallel or series. In parallel, the new timing is half the table time. In series, the new timing is double the table time.
5 ms (−0.026%) = 100 nF and 45.5 kΩ : (two 91 kΩ resistors in parallel)
10 ms (−0.026%) = 100 nF and 91 kΩ : (values from table)
20 ms (−0.026%)= 100 nF and 182 kΩ : (two 91 kΩ resistors in series)
Bistable [ edit ]
Schematic of a 555 in bistable flip-flop mode. High-value pull-up resistors should be added to the two inputs.
Inverted SR flip-flop symbol (without /Q) is similar to circuit on right
In bistable mode, the 555 timer acts as an SR flip-flop. The trigger and reset inputs are held high via pull-up resistors while the threshold input is grounded. Thus configured, pulling the trigger momentarily to ground acts as a "set" and transitions the output pin to V CC (high state). Pulling the reset input to ground acts as a "reset" and transitions the output pin to ground (low state). No timing capacitors are required in a bistable configuration. The discharge pin is left unconnected or may be used as an open-collector output.[28]
Schmitt trigger [ edit ]
Schematic of a 555 in bistable Schmitt trigger mode. Example values R1 and R2 = 100 kΩ, C = 10 nF.
Schmitt trigger inverter gate (lower symbol) is similar to circuit on right
A 555 timer can be used to create a Schmitt trigger inverter gate which converts a noisy input into a clean digital output. The input signal should be connected through a series capacitor, which then connects to the trigger and threshold pins. A resistor divider, from V CC to GND, is connected to the previous tied pins. The reset pin is tied to V CC .
Packages [ edit ]
[1] Texas Instruments NE555 in DIP -8 and SO-8 packages
In 1972, Signetics originally released the 555 timer in DIP-8 and TO5-8 metal can packages, and the 556 timer was released in a DIP-14 package.[4]
In 2006, the dual 556 timer was available in through-hole packages as DIP-14 (2.54 mm pitch),[21] and surface-mount packages as SO-14 (1.27 mm pitch) and SSOP-14 (0.65 mm pitch).
In 2012, the 555 was available in through-hole packages as DIP-8 (2.54 mm pitch),[29] and surface-mount packages as SO-8 (1.27 mm pitch), SSOP-8 / TSSOP-8 / VSSOP-8 (0.65 mm pitch), BGA (0.5 mm pitch).[1]
The MIC1555 is a CMOS 555-type timer with three fewer pins available in SOT23-5 (0.95 mm pitch) surface-mount package.[30]
Specifications [ edit ]
These specifications apply to the original bipolar NE555. Other 555 timers can have different specifications depending on the grade (industrial, military, medical, etc.).
Part number NE555 IC Process Bipolar Supply voltage (V CC ) 4.5 to 16 V Supply current (V CC = +5 V) 3 to 6 mA Supply current (V CC = +15 V) 10 to 15 mA Output current (maximum) 200 mA Maximum Power dissipation 600 mW Power consumption (minimum operating) 30 mW @ 5 V,
225 mW @ 15 V Operating temperature 0 to 70 °C
Derivatives [ edit ]
Numerous companies have manufactured one or more variants of the 555, 556, 558 timers over the past decades, under many different part numbers. The following is a partial list:
Table notes
All information in the above table was pulled from references in the datasheet column, except where denoted below.
For the "Total timers" column, a "*" denotes parts that are missing 555 timer features.
For the " I q " column, a 5-volt supply was chosen as a common voltage to make it easier to compare. The value for Signetics NE558 is an estimate because NE558 datasheets don't state I q at 5 V. [2] The value listed in this table was estimated by comparing the 5 V to 15 V ratio of other bipolar datasheets, then derating the 15 V parameter for the NE558 part, which is denoted by the "*".
" column, a 5-volt supply was chosen as a common voltage to make it easier to compare. The value for Signetics NE558 is an estimate because NE558 datasheets don't state at 5 V. The value listed in this table was estimated by comparing the 5 V to 15 V ratio of other bipolar datasheets, then derating the 15 V parameter for the NE558 part, which is denoted by the "*". For the "Frequency max." column, a "*" denotes values that may not be the actual maximum frequency limit of the part. The MIC1555 datasheet discusses limitations from 1 to 5 MHz. [30] Though most bipolar timers don't state the maximum frequency in their datasheets, they all have a maximum frequency limitation of hundreds of kHz across their full temperature range. Section 8.1 of the Texas Instruments NE555 datasheet [1] states a value of 100 kHz, and their website shows a value of 100 kHz in timer comparison tables. Signetics App Note 170 states that most devices will oscillate up to 1 MHz; however, when considering temperature stability, it should be limited to about 500 kHz. [2] The application note from HFO mentions that at higher supply voltages the maximum power dissipation of the circuit might limit the operating frequency, as the supply current increases with frequency. [42]
Though most bipolar timers don't state the maximum frequency in their datasheets, they all have a maximum frequency limitation of hundreds of kHz across their full temperature range. Section 8.1 of the Texas Instruments NE555 datasheet states a value of 100 kHz, and their website shows a value of 100 kHz in timer comparison tables. Signetics App Note 170 states that most devices will oscillate up to 1 MHz; however, when considering temperature stability, it should be limited to about 500 kHz. The application note from HFO mentions that at higher supply voltages the maximum power dissipation of the circuit might limit the operating frequency, as the supply current increases with frequency. For the "Manufacturer" column, the following associates historical 555 timer manufacturers to current company names. Fairchild Semiconductor was sold to ON Semiconductor in 2016. [43] ON Semiconductor was founded in 1999 as a spinoff of Motorola Semiconductor Components Group. [44] The MC1455 started as a Motorola product. Intersil was sold to Renesas Electronics in 2017. [45] The ICM7555 and ICM7556 started as Intersil products. Micrel was sold to Microchip Technology in 2015. [46] The MIC1555 started as a Micrel product. National Semiconductor was sold to Texas Instruments in 2011. [47] The LM555 and LM556 started as a National Semiconductor products. Signetics was sold to Philips Semiconductor in 1975, later to NXP Semiconductors in 2006. [48] Zetex Semiconductors was sold to Diodes Incorporated in 2008. [49] The ZSCT1555 started as a Zetex product. [50]
556 dual timer [ edit ]
Die of a NE556 dual timer manufactured by STMicroelectronics
[21][16] Pinout of 556 dual timer
The dual version is called 556. It features two complete 555 timers in a 14-pin package; only the two power-supply pins are shared between the two timers.[21][16] In 2020, the bipolar version was available as the NE556,[21] and the CMOS versions were available as the Intersil ICM7556 and Texas Instruments TLC556 and TLC552. See derivatives table in this article.[17][40][39]
558 quad timer [ edit ]
Die of a NE558 quad timer manufactured by Signetics
[2] Pinout of 558 quad timer
[2] 558 internal block diagram. It is different from 555 and 556 timers.
The quad version is called 558 and has four reduced-functionality timers in a 16-pin package designed primarily for "monostable multivibrator" applications.[51][2] By 2014, many versions of 16-pin NE558 have become obsolete.[52]
Partial list of differences between 558 and 555 chips:[2][52]
One V CC and one GND, similar to 556 chip.
and one GND, similar to 556 chip. Four "Reset" are tied together internally to one external pin (558).
Four "Control Voltage" are tied together internally to one external pin (558).
Four "Triggers" are falling-edge sensitive (558), instead of level sensitive (555).
Two resistors in the voltage divider (558), instead of three resistors (555).
One comparator (558), instead of two comparators (555).
Four "Output" are open-collector (O.C.) type (558), instead of push–pull (P.P.) type (555).
See also [ edit ]
References [ edit ]
Further reading [ edit ]
Books
Books with timer chapters
Datasheets
555 Timer Tutorial: Pin Diagram, Specifications & Features
The 555 timer IC is an integral part of electronics projects. Be it a simple project involving a single 8-bit micro-controller and some peripherals or a complex one involving system on chips (SoCs), 555 timer working is involved. These provide time delays, as an oscillator and as a flip-flop element among other applications.
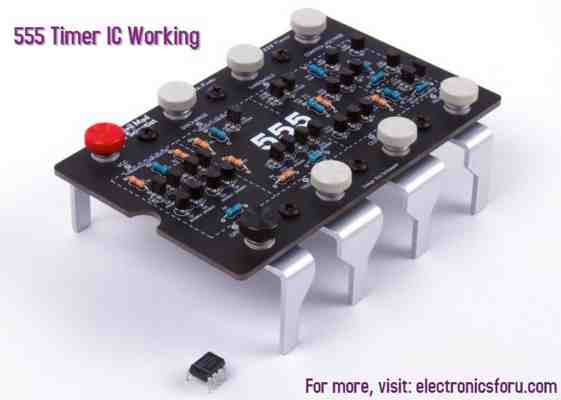
Introduced in 1971 by the American company Signetics, the 555 is still in widespread use due to its low price, ease of use and stability. It is made by many companies in the original bipolar and low-power CMOS types. According to an estimate, a billion units were manufactured back in the year 2003 alone.
Depending on the manufacturer, the standard 555 timer package includes 25 transistors, 2 diodes and 15 resistors on a silicon chip installed in an 8-pin mini dual-in-line package (DIP-8). Variants consist of combining multiple chips on one board. However, 555 is still the most popular. Let us look at the pin diagram to have an idea about the timer Integrated Circuit (IC) before we talk about 555 timer working.
Pin diagram and description
Pin Name Purpose 1 GND Ground reference voltage, low level (0 V) 2 TRIG The OUT pin goes high and a timing interval starts when this input falls below 1/2 of CTRL voltage (which is typically 1/3 Vcc, CTRL being 2/3 Vcc by default if CTRL is left open). In other words, OUT is high as long as the trigger low. Output of the timer totally depends upon the amplitude of the external trigger voltage applied to this pin. 3 OUT This output is driven to approximately 1.7 V below + V cc , or to GND. 4 RESET A timing interval may be reset by driving this input to GND, but the timing does not begin again until RESET rises above approximately 0.7 volts. Overrides TRIG which overrides threshold. 5 CTRL Provides “control” access to the internal voltage divider (by default, 2/3 Vcc). 6 THR The timing (OUT high) interval ends when the voltage at threshold is greater than that at CTRL (2/3 Vcc if CTRL is open). 7 DIS Open collector output which may discharge a capacitor between intervals. In phase with output. 8 V cc Positive supply voltage, which is usually between 3 and 15 V depending on the variation.
Some important features of the 555 timer:
555 timer is used in almost every electronic circuit today. For a 555 timer working as a flip flop or as a multi-vibrator, it has a particular set of configurations. Some of the major features of the 555 timer would be,
It operates from a wide range of power ranging from +5 Volts to +18 Volts supply voltage.
Sinking or sourcing 200 mA of load current.
The external components should be selected properly so that the timing intervals can be made into several minutes along with the frequencies exceeding several hundred kilohertz.
The output pin of a 555 timer can drive a transistor-transistor logic (TTL) due to its high current output.
It has a temperature stability of 50 parts per million (ppm) per degree Celsius change in temperature which is equivalent to 0.005 %/ °C.
The duty cycle of the timer is adjustable.
Also, the maximum power dissipation per package is 600 mW and its trigger pulse and reset inputs has logic compatibility.
555 timer working
The 555 generally operates in 3 modes:
A-stable Mono-stable Bi-stable modes.
Astable mode
This means there will be no stable level at the output. So the output will be swinging between high and low. This character of unstable output is used as a clock or square wave output for many applications.
Mono-stable mode
This configuration consists of one stable and one unstable state. The stable state can be chosen either high or low by the user. If the stable output is set at high (1), the output of the timer is high (1). At the application of an interrupt, the timer output turns low (0). Since the low state is unstable it goes to high (1) automatically after the interrupt passes. Similar is the case for a low stable monostable mode.
Bi-stable mode
In bistable mode, both the output states are stable. At each interrupt, the output changes from low (0) to high (1) and vice versa, and stays there. For example, if we have a high (1) output, it will go low(0) once it receives an interrupt and stays low (0) till the next interrupt changes the status.
This data sheet should provide an insight into the specifics: 555 Timer IC
The below video from Skinny R&D gives an insight into 555 timer as well.
Also, let us know if Jason explained the 555 timer working to your liking.
More tutorials available in the learning corner.
This article was first published on 4th June 2017 and was recently updated on 19th January 2022.
A 555 Timer IC Tutorial
© by Tony van Roon
Ron Harrison
T he 555 timer IC was first introduced arround 1971 by the Signetics Corporation as the SE555/NE555 and was called "The IC Time Machine" and was also the very first and only commercial timer ic available. It provided circuit designers and hobby tinkerers with a relatively cheap, stable, and user-friendly integrated circuit for both monostable and astable applications. Since this device was first made commercially available, a myrad of novel and unique circuits have been developed and presented in several trade, professional, and hobby publications. The past ten years some manufacturers stopped making these timers because of competition or other reasons. Yet other companies, like NTE (a subdivision of Philips) picked up where some left off.
T his primer is about this fantastic timer which is after 30 years still very popular and used in many schematics. Although these days the CMOS version of this IC, like the Motorola MC1455, is mostly used, the regular type is still available, however there have been many improvements and variations in the circuitry. But all types are pin-for-pin plug compatible. Myself, every time I see this 555 timer used in advanced and high-tech electronic circuits, I'm amazed. It is just incredible.
I n this tutorial I will show you what exactly the 555 timer is and how to properly use it by itself or in combination with other solid state devices without the requirement of an engineering degree. This timer uses a maze of transistors, diodes and resistors and for this complex reason I will use a more simplified (but accurate) block diagram to explain the internal organizations of the 555. So, lets start slowly and build it up from there.
The first type-number, in Table 1 on the left, represents the type which was/is preferred for military applications which have somewhat improved electrical and thermal characteristics over their commercial counterparts, but also a bit more expensive, and usually metal-can or ceramic casing. This is analogous to the 5400/7400 series convention for TTL integrated circuits.
T he 555, in fig. 1 and fig. 2 above, come in two packages, either the round metal-can called the 'T' package or the more familiar 8-pin DIP 'V' package. About 20-years ago the metal-can type was pretty much the standard (SE/NE types). The 556 timer is a dual 555 version and comes in a 14-pin DIP package, the 558 is a quad version with four 555's also in a 14 pin DIP case.
Inside the 555 timer, at fig. 3, are the equivalent of over 20 transistors, 15 resistors, and 2 diodes, depending of the manufacturer. The equivalent circuit, in block diagram, providing the functions of control, triggering, level sensing or comparison, discharge, and power output. Some of the more attractive features of the 555 timer are: Supply voltage between 4.5 and 18 volt, supply current 3 to 6 mA, and a Rise/Fall time of 100 nSec. It can also withstand quite a bit of abuse.
The Threshold current determine the maximum value of Ra + Rb. For 15 volt operation the maximum total resistance for R (Ra +Rb) is 20 Mega-ohm.
T he supply current, when the output is 'high', is typically 1 milli-amp (mA) or less. The initial monostable timing accuracy is typically within 1% of its calculated value, and exhibits negligble (0.1%/V) drift with supply voltage. Thus longterm supply variations can be ignored, and the temperature variation is only 50ppm/°C (0.005%/°C).
A ll IC timers rely upon an external capacitor to determine the off-on time intervals of the output pulses. As you recall from your study of basic electronics, it takes a finite period of time for a capacitor (C) to charge or discharge through a resistor (R). Those times are clearly defined and can be calculated given the values of resistance and capacitance.
T he basic RC charging circuit is shown in fig. 4. Assume that the capacitor is initially discharged. When the switch is closed, the capacitor begins to charge through the resistor. The voltage across the capacitor rises from zero up to the value of the applied DC voltage. The charge curve for the circuit is shown in fig. 6. The time that it takes for the capacitor to charge to 63.7% of the applied voltage is known as the time constant (t). That time can be calculated with the simple expression:
t = R X C
A ssume a resistor value of 1 MegaOhm and a capacitor value of 1uF (micro-Farad). The time constant in that case is:
t = 1,000,000 X 0.000001 = 1 second
A ssume further that the applied voltage is 6 volts. That means that it will take one time constant for the voltage across the capacitor to reach 63.2% of the applied voltage. Therefore, the capacitor charges to approximately 3.8 volts in one second.
Fig. 4-1, Change in the input pulse frequency allows completion of the timing cycle. As a general rule, the monostable 'ON' time is set approximately 1/3 longer than the expected time between triggering pulses. Such a circuit is also known as a 'Missing Pulse Detector'.
L ooking at the curve in fig. 6. you can see that it takes approximately 5 complete time constants for the capacitor to charge to amost the applied voltage. It would take about 5 seconds for the voltage on the capacitor to rise to approximately the full 6-volts.
Definition of Pin Functions:
Pin 1 (Ground):
Pin 2 (Trigger):
Pin 3 (Output):
Fig. 3.
Pin 4 (Reset):
Pin 5 (Control Voltage):
Pin 6 (Threshold):
Pin 7 (Discharge):
Pin 8 (V +):
Try the simple 555 testing-circuit of Fig. 5. to get you going, and test all your 555 timer ic's. I build several for friends and family. I bring my own tester to ham-fests and what not to instantly do a check and see if they are oscillating. Or use as a trouble shooter in 555 based circuits. This tester will quickly tell you if the timer is functional or not. Although not foolproof, it will tell if the 555 is shorted or oscillating. If both Led's are flashing the timer is most likely in good working order. If one or both Led's are either off or on solid the timer is defective. Simple huh?
The capacitor slows down as it charges, and in actual fact never reaches the full supply voltage. That being the case, the maximum charge it receives in the timing circuit (66.6% of the supply voltage) is a little over the charge received after a time constant (63.2%).
The capacitor slows down as it discharges, and never quite reaches the ground potential. That means the minimum voltage it operates at must be greater than zero. Timing circuit is 63.2% of the supply voltage.
The discharge of a capacitor also takes time and we can shorten the amount of time by decreasing resistance (R) to the flow of current.
O perating Modes: The 555 timer has two basic operational modes: one shot and astable. In the one-shot mode, the 555 acts like a monostable multivibrator. A monostable is said to have a single stable state--that is the off state. Whenever it is triggered by an input pulse, the monostable switches to its temporary state. It remains in that state for a period of time determined by an RC network. It then returns to its stable state. In other words, the monostable circuit generates a single pulse of a fixed time duration each time it receives and input trigger pulse. Thus the name one-shot. One-shot multivibrators are used for turning some circuit or external component on or off for a specific length of time. It is also used to generate delays. When multiple one-shots are cascaded, a variety of sequential timing pulses can be generated. Those pulses will allow you to time and sequence a number of related operations.
The other basic operational mode of the 555 is as and astable multivibrator. An astable multivibrator is simply and oscillator. The astable multivibrator generates a continuous stream of rectangular off-on pulses that switch between two voltage levels. The frequency of the pulses and their duty cycle are dependent upon the RC network values.
O ne-Shot Operation: Fig. 4 shows the basic circuit of the 555 connected as a monostable multivibrator. An external RC network is connected between the supply voltage and ground. The junction of the resistor and capacitor is connected to the threshold input which is the input to the upper comparator. The internal discharge transistor is also connected to the junction of the resistor and the capacitor. An input trigger pulse is applied to the trigger input, which is the input to the lower comparator.
With that circuit configuration, the control flip-flop is initially reset. Therefore, the output voltage is near zero volts. The signal from the control flip-flop causes T1 to conduct and act as a short circuit across the external capacitor. For that reason, the capacitor cannot charge. During that time, the input to the upper comparator is near zero volts causing the comparator output to keep the control flip-flop reset.
Notice how the monostable continues to output its pulse regardless of the inputs swing back up. That is because the output is only triggered by the input pulse, the output actually depends on the capacitor charge.
Monostable Mode:
The 555 in fig. 9a is shown here in it's utmost basic mode of operation; as a triggered monostable. One immediate observation is the extreme simplicity of this circuit. Only two components to make up a timer, a capacitor and a resistor. And for noise immunity maybe a capacitor on pin 5. Due to the internal latching mechanism of the 555, the timer will always time-out once triggered, regardless of any subsequent noise (such as bounce) on the input trigger (pin 2). This is a great asset in interfacing the 555 with noisy sources. Just in case you don't know what 'bounce' is: bounce is a type of fast, short term noise caused by a switch, relay, etc. and then picked up by the input pin.
The trigger input is initially high (about 1/3 of +V). When a negative-going trigger pulse is applied to the trigger input (see fig. 9a), the threshold on the lower comparator is exceeded. The lower comparator, therefore, sets the flip-flop. That causes T1 to cut off, acting as an open circuit. The setting of the flip-flop also causes a positive-going output level which is the beginning of the output timing pulse.
The capacitor now begins to charge through the external resistor. As soon as the charge on the capacitor equal 2/3 of the supply voltage, the upper comparator triggers and resets the control flip-flop. That terminates the output pulse which switches back to zero. At this time, T1 again conducts thereby discharging the capacitor. If a negative-going pulse is applied to the reset input while the output pulse is high, it will be terminated immediately as that pulse will reset the flip-flop.
Whenever a trigger pulse is applied to the input, the 555 will generate its single-duration output pulse. Depending upon the values of external resistance and capacitance used, the output timing pulse may be adjusted from approximately one millisecond to as high as on hundred seconds. For time intervals less than approximately 1-millisecond, it is recommended that standard logic one-shots designed for narrow pulses be used instead of a 555 timer. IC timers are normally used where long output pulses are required. In this applicaton, the duration of the output pulse in seconds is approximately equal to:
T = 1.1 x R x C (in seconds)
A stable operation: Figure 9b shows the 555 connected as an astable multivibrator. Both the trigger and threshold inputs (pins 2 and 6) to the two comparators are connected together and to the external capacitor. The capacitor charges toward the supply voltage through the two resistors, R1 and R2. The discharge pin (7) connected to the internal transistor is connected to the junction of those two resistors.
When power is first applied to the circuit, the capacitor will be uncharged, therefore, both the trigger and threshold inputs will be near zero volts (see Fig. 10). The lower comparator sets the control flip-flop causing the output to switch high. That also turns off transistor T1. That allows the capacitor to begin charging through R1 and R2. As soon as the charge on the capacitor reaches 2/3 of the supply voltage, the upper comparator will trigger causing the flip-flop to reset. That causes the output to switch low. Transistor T1 also conducts. The effect of T1 conducting causes resistor R2 to be connected across the external capacitor. Resistor R2 is effectively connected to ground through internal transistor T1. The result of that is that the capacitor now begins to discharge through R2.
The only difference between the single 555, dual 556, and quad 558 (both 14-pin types), is the common power rail. For the rest everything remains the same as the single version, 8-pin 555.
As soon as the voltage across the capacitor reaches 1/3 of the supply voltage, the lower comparator is triggered. That again causes the control flip-flop to set and the output to go high. Transistor T1 cuts off and again the capacitor begins to charge. That cycle continues to repeat with the capacitor alternately charging and discharging, as the comparators cause the flip-flop to be repeatedly set and reset. The resulting output is a continuous stream of rectangular pulses.
The frequency of operation of the astable circuit is dependent upon the values of R1, R2, and C. The frequency can be calculated with the formula:
f = 1/(.693 x C x (R1 + 2 x R2))
f = 1/t
or
t = 1/f
D = t1/t = (R1 + R2) / (R1 + 2R2)
t1 = .693(R1+R2)C
t2 = .693 x R2 x C
Applications:
There are literally thousands of different ways that the 555 can be used in electronic circuits. In almost every case, however, the basic circuit is either a one-shot or an astable.
The application usually requires a specific pulse time duration, operation frequency, and duty-cycle. Additional components may have to be connected to the 555 to interface the device to external circuits or devices.
In the remainder of this experiment, you will build both the one-shot and astable circuits and learn about some of the different kinds of applications that can be implemented. Furthermore, the last page of this document contains 555 examples which you can build and experiment with.
Required Parts:
In addition to a breadboard and a DC powersupply with a voltage in the 5 to 12 volt range, you will need the following components: 555 timer, LED, 2-inch /8 ohm loudspeaker, 150-ohm 1/4 watt resistor, two 10K ohm 1/4 resistors, two 1-Mega ohm 1/2 watt resistors, 10 Mega ohm 1/4 watt resistor, 0.1 uF capacitor, and a 0.68uF capacitor. All parts are available from Radio Shack or Tandy.
Experimental steps:
This circuit is resetable by grounding pin 4, so be sure to have an extra wire at pin 4 ready to test that feature.
1. On your breadboard, wire the one-shot circuit as shown in figure 11. 2. Apply power to the circuit. If you have a standard 5 volt logic supply, use it for convenience. You may use any voltage between 5 and 15 volts with a 555 timer. You can also run the circuit from battery power. A standard 9-volt battery will work perfectly. With the power connected, note the status of the LED: is it on or off? ________________ 3. Connect a short piece of hook-up wire to the trigger input line on pin 2. Momentarily, touch that wire to ground. Remove it quickly. That will create a pulse at the trigger input. Note and record the state of LED: _____________________ 4. Continue to observe the LED and note any change in the output state after a period of time. What is the state? ______________ 5. When you trigger the one-shot, time the duration of the output pulse with a stopwatch or the seconds hand on your watch. To do that, the instant that you trigger the one-shot by touching the wire to ground, immediately start your stopwatch or make note of the seconds hand on your watch. Trigger the one-shot and time the ouput pulse. Write in the approximate value of the pulse-duration: ______________________ 6. Using the values of external resistor and capacitor values in Fig. 11 and the time interval formula for a one-shot, calculate the output-pulse duration. What is your value? _____________________ 7. Compare your calculated and timed values of output pulses. Explain any discrepencies between your calculated and measured values. Answer: _________________________________________________ 8. Connect a short piece of hook-up wire to pin 4. You will use that as a reset. 9. Trigger the one-shot as indicated previously. Then immediately thouch the reset wire from pin 4 to ground. Note the LED result: _____________ 10. With a DC voltmeter, measure the output voltage at pin 3 during the one shot's off and on states. What are your values? OFF: __________ volts ON: ___________ volts. 11. Replace the 10 MegOhm resistor with a 1 MegOhm resostor and repeat steps 5 and 6. Record your timed and calculated results: Timed: ________ seconds Calculated: _________seconds
If you want to get fancy, after you've completed the experiment you can replace the resistors with potentiometers to build a variable function generator and play with that to learn more.
12. Next you will experiment with astable circuits. First, rewire the circuit so it appears as shown in Fig. 12. 13. Apply power to the circuit and observe the LED. What is happening? Answer: ____________________________________________________ 14. Replace the 10 MegOhm resistor with a 1 MegOhm resistor. Again observe the LED. Is the frequency higher or lower? _________________ 15. Using the forumla given in the tutorial, calculate the oscillation frequency using R1 as 10 MegOhm, and again with R1 as 1 MegOhm, and again with R1 as 10 MegOhm. R2 is 1 MegOhm in both cases. Record your freq's: f = _____________ Hz (R1 = 10 MegOhm) f = _____________ Hz (R1 = 1 MegOhm) 16. Calculate the period, t1 and t2, and the duty-cycle for each resistor value: 10 MegOhm: t = ___________ t1 = ____________ t2 = ____________ 1 MegOhm: t = ___________ t1 = ____________ t2= ____________
Monitoring the timer with a speaker can be amusing if you switch capacitors or resistors to make an organ.
17. Rewire the circuit making R1 and R2 10,000 ohms and C equal to 0.1uF. Use the same circuit in Fig. 12. But, replace the LED and its resistor with a speaker and capacitor as shown in Fig. 13. 18. Apply power to the circuit and note the result: ______________________ 19. Calculate the frequency of the circuit: f = ____________________ Hz 20. If you have an oscilloscope, monitor the output voltage on pin 3. Disconnect the speaker and note the output. Also, observe the capacitor charge and discharge at pin 6 or 2: _____________________________
Review of steps 1 through 20:
t = 1.1 x .68 x 10-6 x 107 = 7.48 seconds
t = 1/f = 1/.176 = 5.66 seconds
D = t1/t = 5.18/5.66 = .915 or 91.5%
D = 0.942/1.41 = 0.67 or 67%
Example Circuits:
Circuits 1 to 10:
Fig. 1, Dark Detector:
Fig. 2, Power Alarm:
Fig. 3 Tilt Switch:
Fig. 4, Electric Eye Alarm:
Fig. 5, Metronome:
Fig. 6, CW Practice Oscillator:
Fig. 7, CW Monitor:
Fig. 8, Ten-Minute Timer:
Fig. 9, Schmitt Trigger:
Fig. 10, Better Timing:
Circuits 11 to 14:
Fig. 11, Two-Tones:
Fig. 12, Recording Beep:
Fig. 13, Coin Toss:
Fig. 14, Logic Probe:
maximum
Vcc, supply voltage: 18V Input voltage (CONT, RESET, THRES, TRIG): Vcc Output current: 225mA (approx) Operating free-air temp. range: NE555........... 0°C - 70°C SA555........... -40°C - 85°C SE555, SE555C... -55°C - 125°C Storage temperature range: -65°C - 150°C Case temperature for 60sec. (FK package): 260°C
Suggested Reading:
1. 555 Timer IC Circuits. Forrest M. Mims III, Engineer's Mini Notebook. Radio Shack Cat. No: 62-5010.
"Create & experiment with pulse generators, oscillators, and time delays."
2. IC Timer Cookbook. Walter G. Jung. Published by Howard W. Sams & Co., Inc. ISBN: 0-672-21932-8.
"A reference 'must' for hobby, technicians, and engineers."
3. The 555 Timer Applications Sourcebook. Howard M. Berlin. Published by Sams Inc. ISBN: 0-672-21538-1.
"Learn how to connect the 555, perform 17 simple experiments."
Copyright © 1995 - 2000 by Tony van Roon
Revised and updated on March 10, 2001
Comments